Astronomers Observe a New Kind of Massive Cosmic Collision for the First Time
By Dan Falk
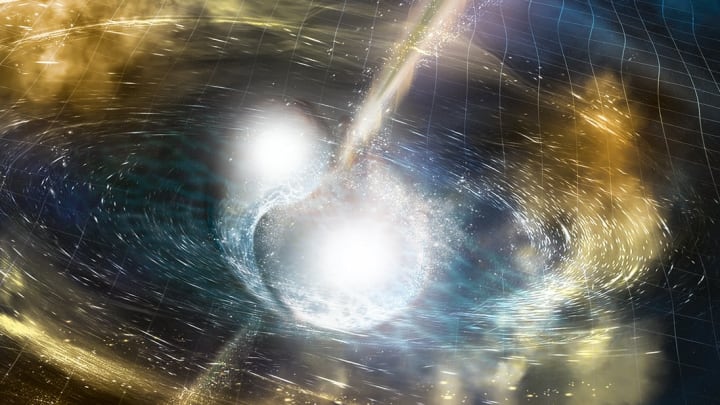
For the first time, astronomers have detected the colossal blast produced by the merger of two neutron stars—and they've recorded it both via the gravitational waves the event produced, as well as the flash of light it emitted.
Physicists believe that the pair of neutron stars—ultra-dense stars formed when a massive star collapses, following a supernova explosion—had been locked in a death spiral just before their final collision and merger. As they spiraled inward, a burst of gravitational waves was released; when they finally smashed together, high-energy electromagnetic radiation known as gamma rays were emitted. In the days that followed, electromagnetic radiation at many other wavelengths—X-rays, ultraviolet, optical, infrared, and radio waves—were released. (Imagine all the instruments in an orchestra, from the lowest bassoons to the highest piccolos, playing a short, loud note all at once.)
This is the first time such a collision has been observed, as well as the first time that both kinds of observations—gravitational waves and electromagnetic radiation—have been recorded from the same event, a feat that required co-operation among some 70 different observatories around the world, including ground-based observatories, orbiting telescopes, the U.S. LIGO (Laser Interferometer Gravitational-Wave Observatory), and European Virgo gravitational wave detectors.
"For me, it feels like the dawning of a next era in astrophysics," Julie McEnery, project scientist for NASA's Fermi Gamma-ray Space Telescope, one of the first instruments to record the burst of energy from the cosmic collision, tells Mental Floss. "With this observation, we've connected these new gravitational wave observations to the rest of the observations that we've been doing in astrophysics for a very long time."
A BREAKTHROUGH ON SEVERAL FRONTS
The observations represent a breakthrough on several fronts. Until now, the only events detected via gravitational waves have been mergers of black holes; with these new results, it seems likely that gravitational wave technology—which is still in its infancy—will open many new phenomena to scientific scrutiny. At the same time, very little was known about the physics of neutron stars—especially their violent, final moments—until now. The observations are also shedding new light on the origin of gamma-ray bursts (GRBs)—extremely energetic explosions seen in distant galaxies. As well, the research may offer clues as to how the heavier elements, such as gold, platinum, and uranium, formed.
Astronomers around the world are thrilled by the latest findings, as today's flurry of excitement attests. The LIGO-Virgo results are being published today in the journal Physical Review Letters; further articles are due to be published in other journals, including Nature and Science, in the weeks ahead. Scientists also described the findings today at press briefings hosted by the National Science Foundation (the agency that funds LIGO) in Washington, and at the headquarters of the European Southern Observatory in Garching, Germany.
(Rumors of the breakthrough had been swirling for weeks; in August, astronomer J. Craig Wheeler of the University of Texas at Austin tweeted, "New LIGO. Source with optical counterpart. Blow your sox off!" He and another scientist who tweeted have since apologized for doing so prematurely, but this morning, minutes after the news officially broke, Wheeler tweeted, "Socks off!")
The neutron star merger happened in a galaxy known as NGC 4993, located some 130 million light years from our own Milky Way, in the direction of the southern constellation Hydra.
Gravitational wave astronomy is barely a year and a half old. The first detection of gravitational waves—physicists describe them as ripples in space-time—came in fall 2015, when the signal from a pair of merging black holes was recorded by the LIGO detectors. The discovery was announced in February 2016 to great fanfare, and was honored with this year's Nobel Prize in Physics. Virgo, a European gravitational wave detector, went online in 2007 and was upgraded last year; together, they allow astronomers to accurately pin down the location of gravitational wave sources for the first time. The addition of Virgo also allows for a greater sensitivity than LIGO could achieve on its own.
LIGO previously recorded four different instances of colliding black holes—objects with masses between seven times the mass of the Sun and a bit less than 40 times the mass of the Sun. This new signal was weaker than that produced by the black holes, but also lasted longer, persisting for about 100 seconds; the data suggested the objects were too small to be black holes, but instead were neutron stars, with masses of about 1.1 and 1.6 times the Sun's mass. (In spite of their heft, neutron stars are tiny, with diameters of only a dozen or so miles.) Another key difference is that while black hole collisions can be detected only via gravitational waves—black holes are black, after all—neutron star collisions can actually be seen.
"EXACTLY WHAT WE'D HOPE TO SEE"
When the gravitational wave signal was recorded, on the morning of August 17, observatories around the world were notified and began scanning the sky in search of an optical counterpart. Even before the LIGO bulletin went out, however, the orbiting Fermi telescope, which can receive high-energy gamma rays from all directions in the sky at once, had caught something, receiving a signal less than two seconds after the gravitational wave signal tripped the LIGO detectors. This was presumed to be a gamma-ray burst, an explosion of gamma rays seen in deep space. Astronomers had recorded such bursts sporadically since the 1960s; however, their physical cause was never certain. Merging neutron stars had been a suggested culprit for at least some of these explosions.
"This is exactly what we'd hoped to see," says McEnery. "A gamma ray burst requires a colossal release of energy, and one of the hypotheses for what powers at least some of them—the ones that have durations of less than two seconds—was the merger of two neutron stars … We had hoped that we would see a gamma ray burst and a gravitational wave signal together, so it's fantastic to finally actually do this."
With preliminary data from LIGO and Virgo, combined with the Fermi data, scientists could tell with reasonable precision what direction in the sky the signal had come from—and dozens of telescopes at observatories around the world, including the U.S. Gemini telescopes, the European Very Large Telescope, and the Hubble Space Telescope, were quickly re-aimed toward Hydra, in the direction of reported signal.
The telescopes at the Las Campanas Observatory in Chile were well-placed for getting a first look—because the bulletin arrived in the morning, however, they had to wait until the sun dropped below the horizon.
"We had about eight to 10 hours, until sunset in Chile, to prepare for this," Maria Drout, an astronomer at the Carnegie Observatories in in Pasadena, California, which runs the Las Campanas telescopes, tells Mental Floss. She was connected by Skype to the astronomers in the control rooms of three different telescopes at Las Campanas, as they prepared to train their telescopes at the target region. "Usually you prepare a month in advance for an observing run on these telescopes, but this was all happening in a few hours," Drout says. She and her colleagues prepared a target list of about 100 galaxies, but less than one-tenth of the way through the list, by luck, they found it: a tiny blip of light in NGC 4993 that wasn't visible on archival images of the same galaxy. (It was the 1-meter Swope telescope that snagged the first images.)
A NEW ERA OF ASTROPHYSICS
When a new star-like object in a distant galaxy is spotted, a typical first guess is that it's a supernova (an exploding star). But this new object was changing very rapidly, growing 100 times dimmer over just a few days while also quickly becoming redder—which supernovae don't do, explains Drout, who is cross-appointed at the Dunlap Institute for Astronomy and Astrophysics at the University of Toronto. "We ended up following it for three weeks or so, and by the end, it was very clear that this [neutron star merger] was what we were looking at," she says.
The researchers say they can't be sure if the resulting object was another, larger neutron star, or whether it would have been so massive that it would have collapsed into a black hole.
As exciting as the original detection of gravitational waves last year was, Drout is looking forward to a new era in which both gravitational waves and traditional telescopes can be used to study the same objects. "We can learn a lot more about these types of extreme systems that exist in the universe, by coupling the two together," she says.
The detection shows that "gravitational wave science is moving from being a physics experiment to being a tool for astronomers," Marcia Rieke, an astronomer at the University of Arizona who is not involved in the current research, tells Mental Floss. "So I think it's a pretty big deal."
Physicists are also learning something new about the origin of the heaviest elements in the periodic table. For many years, these were thought to arise from supernova explosions, but spectroscopic data from the newly observed neutron star merger (in which light is broken up into its component colors) suggests that such explosion produce enormous quantities of heavy elements—including enough gold to put Fort Knox to shame. (The blast is believed to have created some 200 Earth-masses of gold, the scientists say.) "It's telling us that most of the gold that we know about is produced in these mergers, and not in supernovae," McEnery says.
Editor's note: This post has been updated.